Introduction
A few weeks ago I posted an article on consciousness. This post is a continuation of my series on cognition, focusing at the other end of the spectrum on the concept of “minimal intelligence.” When we think of intelligence, we typically picture the human brain or the minds of other mammals. However, the natural world is full of examples of minimal intelligence, frequently underestimated cognitive processes that challenge this neuron-centric perspective. Every organism inhabits a complex environment and must be capable of gathering information, processing it, and making decisions to survive. These cognitive activities are present across all forms of life, from the simplest to the most complex, tracing back to our earliest ancestors.
In the words of biologist and philosopher Humberto Maturana, “Living systems are cognitive systems and living as a process is a process of cognition. This statement is valid for all organisms with and without a nervous system” (Maturana 1970).
This blog post will explore the cognitive behaviors exhibited by such non-neural systems. We’ll focus on the intelligent actions of single-celled organisms, plants, and slime molds. We will also discuss the sophisticated intra- and inter-cellular processes of advanced organisms. These examples offer to expand our appreciation of the diverse manifestations of minimal intelligence that permeate life.
Cellular Minimal Intelligence
Minimal intelligence in Bacteria
Single-celled organisms, despite their simplicity, exhibit remarkably intelligent behaviors. Even bacteria exhibit cognitive behaviors such as decision making, learning, and memory. One significant mechanism mediating bacterial cognitive processes is the two-component system. These protein complexes regulate activities ranging from sporulation to pathogenesis in response to various stimuli. In some cases, different versions of these protein signaling systems may collectively form a “phosphoneural network,” integrating diverse types of environmental information to produce a coordinated response (Hellingwerf 2005).
For example, in flagellated bacteria like E. coli, the CheA-CheY two-component system mediates chemotaxis behavior. This system responds to output from clusters of approximately 10,000 chemosensors, sometimes referred to as “nanobrains.” Information is integrated from multiple parameters, including various nutrients, temperature, pH, salts, and bacterial signaling molecules. Additionally, the methylation state of these sensors allows the bacteria to “remember” previous encounters and adapt their behavior accordingly (Mikhail et al. 2002).
Bacteria also exhibit an “awareness” of their own species. They can communicate in various ways and alter their behavior once a quorum is reached. Moreover, under starvation conditions, some bacteria self-organize into highly ordered structures capable of collective sensing, distributed information processing, and coordinated gene regulation. Remarkably, these abilities are facilitated by communication through voltage-gated potassium channels. Homologs of these proteins are used to propagate action potentials in modern neurons. It is likely that these bacterial proteins served as evolutionary precursors for this function (Prindle et al. 2015).
Minimal Intelligence in Protists
The realm of protists is also replete with examples of cognitive behaviors, challenging our understanding of intelligence in simple organisms. Among these are the learning and memory of paramecia, the complex responses of Stentor, and the architectural skills and cooperative hunting strategies of certain amoebae.
Paramecium: Learning and Memory
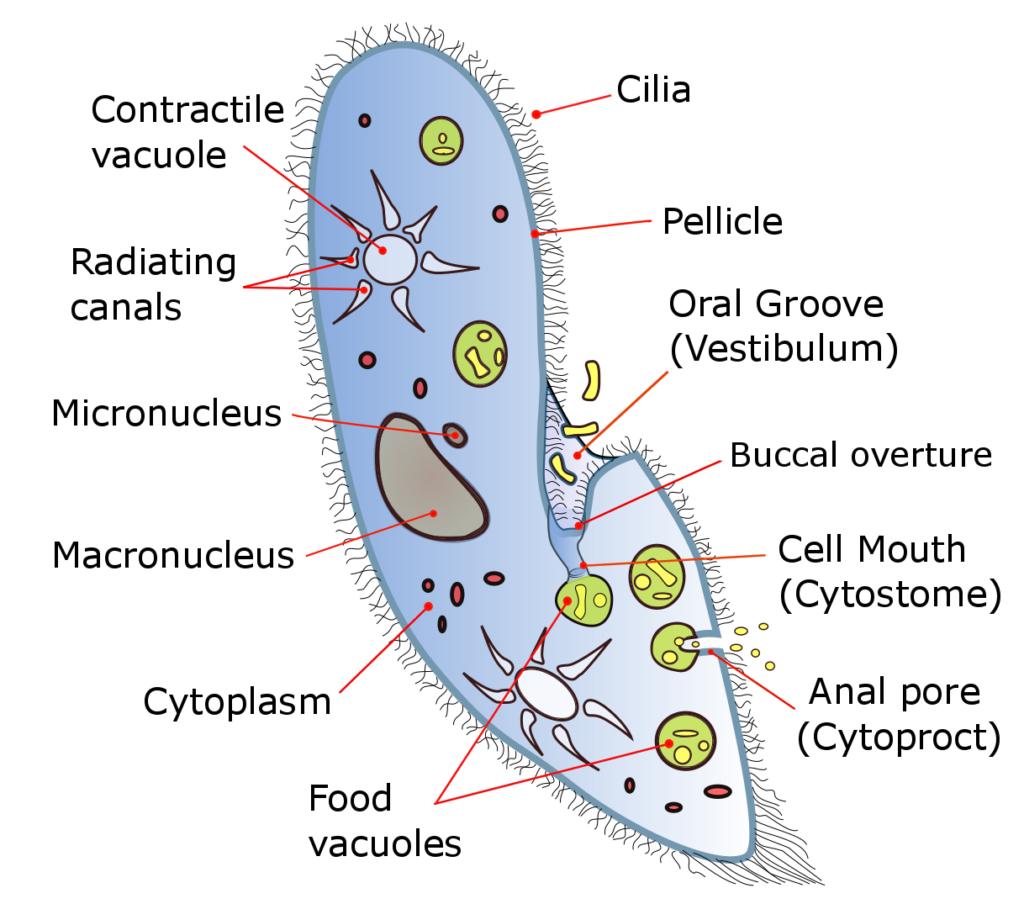
Paramecium exhibits a sophisticated behavioral repertoire to navigate its sensory-rich environment. It finds food by detecting chemicals from decaying plants and other paramecia, moves toward the water surface via gravitaxis, and avoids obstacles through mechanosensitivity. Further, it resists water currents with rheotaxis, avoids bright light, and steers clear of hot and cold water. What is even more impressive, however, is that these organisms exhibit a capacity for learning and memory.
One example of minimal intelligence that has been consistently reproduced is tube escape learning. In this experiment, a single Paramecium is placed in a drop of water. Then, a thin tube is lowered into it, drawing the organism into the tube by capillary action. Initially, the Paramecium takes about 30 seconds to escape from the bottom of the tube. With repeated trials, however, the escape time decreases to around 15 seconds. This quicker escape behavior persists for at least two hours, suggesting a form of learning rather than simple adaptation.
Paramecium have also been trained to react to sounds. Initially, they show no response to a tone played by a speaker. However, when the tone is paired with electrical stimulation, the Paramecium reacts with an avoiding behavior. After a few trials, they begin to show an avoiding reaction at the onset of the tone, anticipating the electrical stimulus. This learned behavior demonstrates extinction (the reaction disappears when the tone is presented alone), retention, and specificity (reacting specifically to a 300-Hz or 500-Hz tone).
These remarkable abilities are partly due to possessing many of the same signaling pathways found in neurons. These include calcium signaling pathways, calcium release channels, calmodulin, centrin, calcineurin, SNARE proteins, and cAMP and cGMP-dependent kinases, and a rhodopsin like protein. Due to these similarities, some researchers have referred to Paramecium as a “swimming neuron.”
Stentor: Adaptive Responses
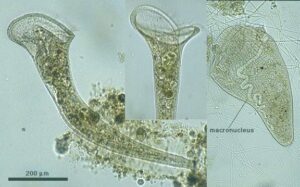
Stentor, a large ciliate, exhibits minimal intelligence in its adaptive behaviors. When exposed to repeated non-lethal stimuli, such as gentle prodding, Stentor initially contracts as a defense mechanism. However, with repeated exposure, it learns to ignore the harmless stimulus, displaying a form of habituation. This ability to modify its behavior based on experience indicates a level of environmental awareness and learning capacity that is quite advanced for a single-celled organism.
Stentor can also engage in decision making. Not only do they display a hierarchy of avoidance behaviors, if the initial response is not effective, they are able to “change their minds”. This suggests a speculative form of cognition.
Minimal Intelligence in Amoebae: Cooperative Hunting and Building Houses
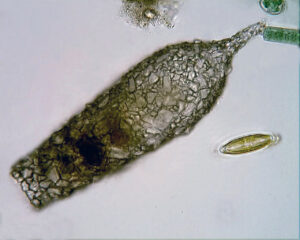
Certain protists such as the testate amoebae exhibit architectural intelligence by constructing protective “houses” known as tests. They make them from sand, diatoms, and other particles. This behavior involves the selection of appropriate materials and their careful assembly into a functional structure. It demonstrates a sophisticated level of spatial awareness and construction ability.
Another amoebae, the cellular slime mold Dictyostelium discoideum, (featured image) displays cooperative hunting strategies that are strikingly sophisticated. When food is scarce, individual amoebae release chemical signals that attract others, leading to the aggregation of thousands of cells.
These aggregated cells form a multicellular structure that moves as a single entity, seeking out food sources. This collective behavior allows them to hunt more effectively and survive in challenging environments. The coordination and communication involved in exemplifies a collective minimal intelligence that enhances their ability to adapt and thrive.
Plasmodial Slime Mold Problem Solving and Learning
The cognitive abilities of slime molds suggest that even simple organisms can engage in, learning, communication, and complex information processing. They expand our understanding of the fundamental principles of intelligence and learning in living systems.
Collective Behavior
The collective intelligence exhibited by slime molds, particularly Physarum polycephalum, allows them to adapt and thrive in their environment. Initially, slime molds exist as individual, amoeba-like cells that move and feed independently. However, when conditions become unfavorable, such as when food becomes scarce, these individual cells aggregate and fuse together to form a larger, multinucleated cell called a plasmodium. (See life cycle diagram below)
When foraging for nutrients, plasmodia employ a decentralized decision-making process. They extend pseudopods in multiple directions, exploring their environment. Upon encountering a nutrient source, the pseudopods carrying the highest concentration of nutrients signal the rest of the organism, guiding the entire plasmodium toward the food. This process is akin to a distributed computing system, where individual parts of the organism work together to achieve a common goal.

Problem-Solving Abilities
One of the most fascinating aspects of slime mold intelligence is their ability to solve complex problems. These talents have been demonstrated by placing plasmodia in environments with scattered food sources. The organisms respond by creating networks that optimize nutrient acquisition while minimizing energy expenditure. Their efficiency is comparable to that seen in railway systems.
Moreover, slime molds can solve a maze for the optimal path to food sources. This behavior suggests an ability to process information and make decisions, akin to problem-solving strategies seen in more complex organisms.
Possibly the most astonishingly these organisms have been recruited to solve complex problems in mathematics. For instance, they are able to solve the Traveling Salesman Problem (TSP). The TSP involves finding the shortest possible route to visit a set of cities and return to the starting point. Despite its simplicity, TSP is computationally challenging and is a classic example of an NP-hard problem in computer science. Slime molds can solve the equivalent problem when tasked with maximizing their body area for nutrient absorption while minimizing the risk of being exposed to aversive light stimuli.
Scientists have even used algorithms inspired by slime molds to effectively map the universe’s “cosmic web”. This web of matter, formed by gravity over billions of years, consists of filaments, tendrils, and voids that connect galaxies like beads on a string. By using a slime-mold-based algorithm, researchers created a detailed map that surpassed human-designed methods in both sensitivity and accuracy. The algorithm was even able to map dark matter with a new level of precision, revealing how the cosmic web influences galaxy formation over time.
Learning, Remembering, and Sharing Information
Research has also shown that Physarum polycephalum also has the ability to learn. In these experiments, slime molds were exposed to periodic shocks, such as cold or dry conditions. Initially, they reacted by altering their growth patterns to avoid the unfavorable conditions. However, after repeated exposures at consistent intervals, the slime molds began to exhibit anticipatory behavior. They would slow their growth or move to a more favorable area just before the expected shock, indicating that they had “learned” the pattern of exposure.
In addition, slime molds are capable of adapting to unfavorable chemicals over time. This form of learning is called habituation. Researchers demonstrated this by challenging slime molds to cross a bridge treated with quinine or caffeine to reach food. Initially the slime molds were reluctant to cross the bridge. After repeated exposure, however, they reduced their crossing time by half.
A separate experiment, shows slime molds are capable of sharing this learned behavior with other slime molds through fusion. A slime mold habituated to salt was fused with a “naive” slime mold. After two to three hours of fusion, the combined slime molds showed no hesitation in crossing a salted bridge, indicating that the habituated slime mold had transferred its learning to the naive one.
Plant Minimal Intelligence
We often perceive plants as passive organisms. Unlike animals, whose actions can be immediate and observable, plant behaviors occur over extended periods. This slow pace makes it challenging for humans to recognize the sophisticated processes at play. As a result, the remarkable intelligence of plants often goes unappreciated because it operates on a timescale different from our own. However, plants exhibit a range of intelligent behavior. These include including navigating their environment, communicating with each other, and learning from their experiences. If you are skeptical, check out this video from the New Yorker narrated by Michael Pollan.
Moreover, plants coordinate their behaviors through a complex, integrated system of signaling, communication, and response mechanisms. This system involves many components, including: long-distance electrical signals similar to those of neurons, the movement of the hormone auxin through specialized vascular tissues, and the production of various chemicals typically associated with animal nervous systems, such as neurotransmitters like acetylcholine, catecholamines, histamines, serotonin, dopamine, melatonin, GABA (gamma-aminobutyric acid), and glutamate.
Memory, Learning, and counting
Research suggests that plants can remember and learn from past experiences. A notable example is the sensitive plant, Mimosa pudica. When touched, its leaves fold up as a defensive response. Studies have shown that after repeated harmless touches, Mimosa pudica learns to keep its leaves open. This behavior indicates a form of learning and memory, demonstrating that plants can modify their responses based on past experiences.
Venus flytraps exhibit similar behavior. This plant has a form of short-term memory that lasts about 30 seconds. If an insect taps the plant’s sensitive hairs only once, the trap remains still. However, if the insect taps again within this half-minute window, the carnivorous plant’s leaves snap shut, ensnaring its prey.
Then, once the trap is closed, the Venus flytrap requires at least three more touches to ensure it has caught prey before activating the genes responsible for producing insect-digesting enzymes. In essence, the plant can count to five.
Communication and Defense
Another area where plants exhibit intelligence, is in their ability to communicate and defend themselves using chemical signals. When herbivores attack certain plants, they release volatile organic compounds (VOCs) into the air. These VOCs serve as distress signals, alerting nearby plants to the presence of a threat.
For instance, when attacked by insects, tomato plants emit VOCs that trigger nearby tomato plants to produce proteinase inhibitors. These inhibitors interfere with the digestive enzymes of the herbivores, making it more difficult for them to digest the plant material (Cappola et al 2017).
Similarly, willow trees attacked by herbivores produce salicylic acid, which travels through the air and signaling neighboring willows. In response, they bolster their defenses by producing chemicals that make their leaves less palatable to herbivores. This form of communication shows that plants can perceive danger and share information to collectively enhance their chances of survival.
Another example occurs when caterpillars attack corn plants. In response they release a specific blend of VOCs that attract parasitic wasps. These wasps lay their eggs inside the caterpillars, ultimately killing them. This indirect defense mechanism not only protects the corn plant but also reduces the caterpillar population in the area.
Plants also activate physical defenses in response to VOC signals. For example, some plants increase the production of trichomes (tiny hair-like structures) on their leaves. This can deter herbivores by making the leaves less palatable or more difficult to eat. These sophisticated mechanisms highlight the complex and adaptive nature of plant intelligence. They ensure not only the survival of individual plants but also the health and stability of plant communities.
The Minimal Intelligence of Plant Roots
Emerging research suggests that plants, particularly their root systems, possess forms of learning, memory and information processing. These behaviors allow them to optimize their growth and responses based on past experiences. The result is enhanced adaptability and resilience in dynamic environments.
Navigation
Plant roots display an impressive ability to integrate information for the navigation of their surroundings. They seek out nutrients and water while avoiding obstacles and harmful substances. A combination of chemical and physical signals drive this behavior. Roots grow towards areas with higher concentrations of nutrients, a process known as chemotropism. They also adjust their growth in response to physical barriers and changes in the soil’s texture. These capabilities demonstrate a sophisticated level of environmental awareness and adaptability.
Memory formation
Plant roots also exhibit the ability to form memories. For instance, when roots encounter a specific nutrient gradient, they may grow toward the higher concentration. If this nutrient becomes scarce over time, the roots may “remember” the previous location and alter their growth direction more efficiently when the nutrient is detected again. This ability to integrate and respond to multiple environmental cues demonstrates a sophisticated level of information processing.
Learning from Stress Responses
Studies have shown that plants subjected to repeated stress can “learn” to optimize their root growth. For instance, roots exposed to periodic drought conditions may alter their growth strategy to develop deeper root systems more rapidly when they encounter similar conditions again. This adaptive behavior indicates that plants are capable of integrating past experiences into their growth strategies, much like memory and learning in animals.
Molecular Mechanisms
The molecular basis of memory and learning in plant roots involves complex signaling pathways and hormonal regulation. Plants use a variety of signaling molecules, such as calcium ions, reactive oxygen species (ROS), and plant hormones like auxins, to process environmental information. Moreover, transient changes the concentrations of these molecules can trigger longer-term physiological changes. These changes may be “recorded” in the form of modified protein activities or epigenetic modifications, which alter gene expression patterns and provide a molecular basis for memory.
Inter- and Intracellular Minimal Intelligence of Multicellular Organisms
Cells of multicellular organisms also exhibit complex decision-making processes and coordinated activities, demonstrating a remarkable form of intracellular intelligence. These processes involve elaborate systems that regulate gene expression and perform essential cellular functions with precision.
Development as an Intelligent Intercellular Process
For instance, the ability to interpret information, make decisions, and adapt to environmental conditions characterizes animal development. Viewing development as intelligent challenges the traditional notion of it as a strictly mechanistic and deterministic sequence driven by a combination of genetic instructions, and the laws of physics and chemistry. Instead, it emphasizes the dynamic, interactive, self-organizing and generative nature of developmental processes, showcasing the intrinsic intelligence of living systems.
During embryogenesis, a single fertilized egg cell divides and differentiates into various cell types, tissues, and organs, forming a fully functional organism. This transformation involves elaborate communication and coordination among cells, mediated by signaling molecules and feedback loops. Cells sense their positions relative to each other and the environment, interpret chemical gradients, and make decisions about their fate based on these cues. For example, the formation of the vertebrate limb requires precise spatial and temporal regulation of gene expression and cell behavior, guided by morphogen gradients that provide positional information to developing cells.
The Minimal Intelligence of Intracellular Systems
Gene Regulatory Networks
GRN Computation
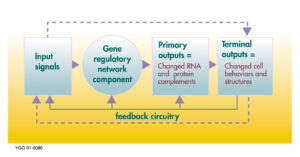
Much of what makes development possible are the non-neural cognitive processes found in the regulatory genomes of metazoans. Computational biologist Sorin Istrail refers these cognitive units as “genomic computers.” They consist of several hundred thousand cis-regulatory modules that govern gene expression, acting as information processors.
These circuits are known as gene regulatory networks, capable of receiving inputs from various sources reflecting the cell’s state. They perform basic logic functions such as AND, OR, and NOT, similar to electronic computers. For example, a cis-regulatory module might activate a gene only when two specific proteins are present (AND), inhibit a gene when a repressor protein is present (NOT), or activate a gene when either of two different signals are received (OR). This combinatorial logic allows cells to integrate multiple signals and make precise decisions about gene expression.
GRN’s In Development
Gene regulatory networks are essential for the spatial and temporal regulation of gene expression during development. They enable cells to respond dynamically to changing environmental conditions and intercellular signals, guiding processes like cell differentiation, tissue formation, and organ development. These networks ensure that genes are turned on and off at the right times and in the right places, facilitating the complex choreography of development.
Embryos also utilize genetically encoded cellular memory to guide cell activity based on prior “experience.” For example, HOX gene expression patterns serve as a form of positional memory, providing cells with an internal representation of their location within the multicellular organism. Additionally, hysteresis in Hedgehog protein signaling—where the response of cells to a stimulus depends on their history of exposure to that stimulus—contributes to this cellular memory, ensuring that cells remember previous signals and respond accordingly in future development stages.
Istrail notes that “the genomic computer controls spatial gene expression in the development of the body plan, and its appearance in remote evolutionary time must be considered to have been a founding requirement for animal-grade life”. This perspective underscores the idea that the complex regulation of gene expression through gene regulatory networks is a fundamental aspect of biological intelligence, driving the emergence and evolution of complex life forms.
By understanding the role of gene regulatory networks in development, we can appreciate the profound intelligence embedded within the genome. These networks exemplify how biological systems process information, make decisions, and adapt to their environment, contributing to the remarkable diversity and complexity of life.
The Spliceosome
Another remarkable example of intracellular intelligence is the spliceosome, a complex molecular machine responsible for RNA splicing. RNA splicing is a process where introns (non-coding regions) are removed from pre-mRNA, and exons (coding regions) are joined together to form mature mRNA. The spliceosome integrates information from various sources to perform this task accurately and efficiently.
The spliceosome consists of multiple small nuclear RNAs (snRNAs) and numerous proteins that work in concert. It recognizes specific sequences within the pre-mRNA that mark the boundaries of introns and exons. The spliceosome’s ability to accurately identify these sequences and execute precise cuts and joins in the RNA molecule is crucial for generating correct and functional mRNA transcripts.
This process involves a high level of coordination and regulation. The spliceosome must respond to various signals and factors that influence splicing decisions. This includes the presence of alternative splicing signals, regulatory proteins, and the overall cellular context. By integrating these inputs, the spliceosome can produce many different mRNA variants from a single gene, a phenomenon known as alternative splicing. This capability allows cells to generate a diverse array of proteins from a limited number of genes, greatly enhancing the complexity and adaptability of the proteome.
Motor Proteins
Motor proteins are another example of molecular machines demonstrating information processing and decision-making behaviors. These proteins, such as kinesin and dynein, transport various cellular components along microtubules. They move cargo to specific locations within the cell, a task requiring precise coordination and directionality. Motor proteins use chemical energy from ATP to “walk” along microtubules, carrying essential materials to where they are needed. During this process, motor proteins must decide the correct path to take and adjust their movements based on the cellular environment. This includes avoiding obstacles and ensuring timely delivery during cell division or intracellular signaling.
Practical Applications of Minimal Intelligence
Bio-Inspired Technologies
Exploring minimal intelligence in non-neural systems can inspire innovative technologies and solutions in various fields. For instance, studying the cognitive behaviors of these systems can lead to advancements in robotics, computing, and artificial intelligence. For example, the way slime molds solve complex problems, such as finding the shortest path through a maze, can inform algorithms for network optimization and route planning. These algorithms can be applied to enhance the efficiency of transportation systems, communication networks, and logistics operations.
In robotics, the decentralized decision-making observed in slime molds and bacterial colonies can inspire the development of swarm robotics. Swarm robots operate based on simple rules and local interactions, mimicking the collective intelligence seen in these organisms. This approach can be used to design robots that work together to achieve tasks like search and rescue, environmental monitoring, and agricultural automation.
Plants’ ability to adapt to their environment and optimize resource use offers insights for sustainable technology development. For example, mimicking the root navigation strategies of plants can lead to the creation of advanced sensors and adaptive systems for soil health monitoring and precision agriculture.
Ecological and Agricultural Innovations
The study of minimal intelligence in plants and other organisms has significant implications for ecology and agriculture. By leveraging the natural adaptive strategies of plants, we can improve crop resilience and sustainability. Additionally, understanding how plants communicate and defend themselves may inspire new pest control and crop protection methods, reducing the reliance on harmful pesticides.
Understanding the communication and signaling processes in plants can also facilitate the development of more efficient and sustainable agricultural practices. For instance, using plant-derived chemicals to trigger defense responses in crops can protect them from pests and diseases without harming the environment. This approach aligns with the principles of integrated pest management, promoting ecological balance and reducing the environmental impact of agriculture.
Knowledge of how plant roots to remember and learn from past experiences also has useful applications. Understanding these processes can help in developing crops that are more resilient to stress conditions such as drought and nutrient deficiency. By breeding or engineering plants with enhanced memory and learning capabilities, it may be possible to create crops that are better able to adapt to changing environmental conditions, improving yield and sustainability.
Theoretical and Philosophical Perspectives on Minimal intelligence
Exploring the intelligence of non-neural systems such as cells, plants, and slime molds invites us to reconsider the very nature of cognition and intelligence. Several theoretical perspectives, such as panpsychism and cognitive biology, provide intriguing frameworks for understanding these phenomena.
Panpsychism and Cognitive Biology
Panpsychism is a philosophical view that posits that consciousness or cognitive qualities are fundamental aspects of all matter, extending beyond humans and animals to include all living organisms and even inanimate objects. According to this perspective, every entity possesses some degree of consciousness or cognitive ability, no matter how rudimentary. This theory challenges the traditional view that intelligence and cognition are exclusive to complex neural systems.
Cognitive biology, on the other hand, is an interdisciplinary field that studies cognition as a fundamental property of life. It seeks to understand how living organisms process information, make decisions, and adapt to their environments, regardless of the presence of a nervous system. Cognitive biology looks at cognitive processes in the context of biological evolution, emphasizing that even the simplest organisms exhibit forms of information processing and decision-making.
These theoretical perspectives broaden the scope of what we consider to be cognitive behavior. By acknowledging that cognition can occur in various forms and at different levels of complexity, we can appreciate the diverse manifestations of intelligence across the biological spectrum.
Philosophical Reflections on Minimal Intelligence
The discovery of cognitive behaviors in non-neural systems also invites profound philosophical reflections on life, consciousness, and intelligence. Traditionally, intelligence and consciousness have been associated with complex neural networks and brains. However, the minimal intelligence observed in plants, slime molds, and microorganisms challenge this neural-centric view and suggests that these traits are fundamental to life.
These insights can reshape our philosophical understanding of consciousness. If cognitive processes are present in simple organisms, it raises questions about the nature and origins of consciousness. Is consciousness an emergent property of complex systems, or is it a more pervasive aspect of life itself? This line of inquiry encourages a more inclusive and holistic view of consciousness, considering it as a spectrum rather than a binary attribute.
Furthermore, recognizing the intelligence of non-neural systems blurs the boundaries between humans and other forms of life. It highlights the interconnectedness and continuity of cognitive abilities across the biological spectrum, fostering a sense of kinship with all living organisms. This perspective can inspire a more compassionate and ethical relationship with the natural world, emphasizing our shared cognitive heritage.
Conclusion
Recognizing minimal intelligence in non-neural systems has profound implications for our understanding of intelligence. It challenges the conventional view that intelligence is solely a product of complex neural networks and suggests that cognitive abilities are more widespread and fundamental than previously thought.
This expanded view prompts us to redefine what it means to be intelligent. Traditional definitions of intelligence often focus on problem-solving, learning, and memory within the context of neural activity. However, the behaviors observed in cells, plants, and slime molds demonstrate that these cognitive processes can also arise in non-neural systems. This realization encourages us to develop more inclusive and nuanced definitions of intelligence that encompass a broader range of biological phenomena.
Moreover, by understanding how simple organisms process information and make decisions, we can uncover the basic principles underlying cognitive behavior. This knowledge can inform various fields, from artificial intelligence and robotics to ecology and agriculture, by providing new models and strategies for problem-solving and adaptation.
Further, this perspective encourages a more holistic view of life and cognition. It suggests that cognitive processes are not confined to the brain but are intrinsic to all living systems. This holistic view can inspire new approaches to studying and interacting with the natural world, fostering a deeper appreciation for the complexity and interconnectedness of life.
Be sure to visit bleedingedgebiology.com next week for another “bleeding edge” topic!
Your Thoughts?
We hope this exploration of minimal intelligence in non-neural systems has expanded your understanding of the remarkable cognitive abilities present in all forms of life. What are your thoughts on the idea that intelligence extends beyond the brain? How do you think these insights could impact our future technologies, agricultural practices, and ethical considerations?
Do you have experiences or examples of non-neural intelligence that you’ve encountered? How do you believe this broader understanding of intelligence could shape our relationship with the natural world?
Leave your comments below and join the conversation! Your insights and questions are valuable as we continue to explore the diverse manifestations of intelligence in life.
Additional Materials
To further explore the fascinating world of non-neuronal intelligence, we recommend the following articles, books, TED Talks, and documentaries. These resources provide in-depth insights and engaging perspectives on the cognitive behaviors of plants, microorganisms, and other non-neural systems.
Articles:
- “‘On Having No Head’: Cognition throughout Biological Systems” F Baluška, M Levin Frontiers in psychology 7 (2016): 902. Discusses current knowledge of the molecular mechanisms at work in non-neuronal cognitive systems, and suggest several hypotheses for future investigation.
- “Remarkable problem-solving ability of unicellular amoeboid organism and its mechanism” Zhu et al. Royal Society Open Science 5.12 (2018): 180396 – Researchers show that the slime mold Physarum polycephalum is able to solve the Traveling Salesman Problem, a combinatorial test with exponentially increasing complexity, in linear time.
- “Organization of the Receptor-Kinase Signaling Array That Regulates Escherichia coli Chemotaxis”– in flagellated bacteria like E. coli, the CheA-CheY two-component system mediates chemotaxis behavior. Levit et al. Journal of Biological Chemistry 277.39 (2002): 36748-36754.This system responds to output from clusters of approximately 10,000 chemosensors, sometimes referred to as “nanobrains.”
- “The Foundations of Plant Intelligence” Trewavas Annals of botany 92.1 (2003): 1-20. A review of plant intelligence.
- “The Regulatory Genome and the Computer” Istrail et al. Developmental Biology 310.2 (2007): 187-195. Considers the operating principles of the genomic computer in comparison to those of the genomic computer.
- “Plant-to-plant communication triggered by systemin primes anti-herbivore resistance in tomato.” Coppola, Mariangela, et al. Scientific reports 7.1 (2017): 15522. Systemin a peptide released in respose to herbivory, triggers the release of VOCs capable of inducing a primed state in neighboring unchallenged plants. Systemin-primed plants were significantly more resistant to herbivorous pests, more attractive to parasitoids and showed an increased response to wounding.
Books:
- “The Light Eaters: How the Unseen World of Plant Intelligence Offers a New Understanding of Life on Earth” by Zoë Schlanger 2024 – The latest scientific research reveals minimal intelligence, creativity, and even heightened awareness in plants, fundamentally transforming our perception of them.
- “Brilliant Green: The Surprising History and Science of Plant Intelligence” by Stefano Mancuso and Alessandra Viola Island Press 2015 – This book delves into the scientific evidence supporting the minimal intelligence of plants, challenging traditional views of plant life.
TED Talks:
- “The Roots of Plant Intelligence” by Stefano Mancuso 2010 – Stefano Mancuso presents fascinating evidence of plant minimal intelligence and the sophisticated behaviors of plant roots.
- “How Bacteria ‘talk’” by Bonnie Bassler 2009 – Bonnie Bassler discusses how bacteria communicate and cooperate, revealing insights into microbial minimal intelligence.
- “What humans can learn from semi-intelligent slime” by Heather Barnett 2014 – Inspired by biological design and self-organizing systems, artist Heather Barnett co-creates with physarum polycephalum, a eukaryotic microorganism that lives in cool, moist areas.
Documentaries
- “Intelligence Without Brains” World Science Festival 2019- An exploration of plants that learn new behaviors and warn their brainless fellows of danger; vines that compete with each other; molds that solve puzzles; and trees that communicate and cooperate through a ‘wood-wide web’ of microscopic mycological fibers.
- “David Attenborough’s Kingdom of Plants“ Britbox 2012- A visually stunning documentary that explores the complex lives of plants, highlighting their adaptive strategies and intelligent behaviors.
- “Plant Neurobiology” Short video by Michael Pollan from the New Yorker 2014 – Michael Pollan discusses how timelapse photography reveals the minimal intelligence of plants.
Your Thoughts?
We hope this exploration of minimal intelligence in non-neural systems has expanded your understanding of the remarkable cognitive abilities present in all forms of life. What are your thoughts on the idea that intelligence extends beyond the brain? How do you think these insights could impact our future technologies, agricultural practices, and ethical considerations?
Do you have experiences or examples of non-neural intelligence that you’ve encountered? How do you believe this broader understanding of intelligence could shape our relationship with the natural world?
Leave your comments below and join the conversation! Your insights and questions are valuable as we continue to explore the diverse manifestations of intelligence in life.