Introduction
When we think of green energy, solar panels, wind turbines, and dams often come to mind. But there’s another piece of the sustainability puzzle you might not know about: microbial energy conversion. This innovative approach uses bacteria to turn organic waste into energy, addressing both waste management and energy production.
As industries expand and the population grows, scalable, eco-friendly energy solutions become more urgent. Microbial energy conversion presents an exciting opportunity. Technologies like anaerobic digestion, microbial fuel cells, and microbial electrolysis cells harness microbes to break down organic matter. This process turns waste into valuable energy resources.
In this post, I’ll explore how these lesser-known microbial processes can help us build a more sustainable future, adding yet another layer to the complex landscape of renewable energy solutions.
Anaerobic Digestion: A Core Component of Microbial Energy Conversion
Definition and Process
Lets start with the basics: Anaerobic digestion is a key process in microbial energy conversion. In the absence of oxygen, bacteria decompose waste such as food scraps, manure, or sewage sludge. This generates biogas, typically composed of 50-70% methane, along with carbon dioxide and trace gases like hydrogen sulfide.
Applications of Anaerobic Digestion in Microbial Energy Conversion
Anaerobic digestion tackles both waste reduction and energy production, making it useful across various sectors. For instance, wastewater treatment plants harness anaerobic digestion for microbial energy conversion, effectively managing sewage sludge.The magic happens as the process produces biogas that can often power the plant itself, helping it achieve energy self-sufficiency.
In agriculture, anaerobic digestion helps manage animal manure by converting it into biogas, which can power farm operations and reduce greenhouse gas emissions. And let’s not forget the digestate, which returns to the soil as a nutrient boost, enhancing soil health.
Even in landfills, anaerobic digestion comes into play as a component of microbial energy conversion. As organic waste breaks down, it naturally generates methane. Landfills can capture this methane through controlled gas recovery systems, converting it into energy. This process significantly reduces methane emissions, turning a potential environmental hazard into a useful resource.
Scalability and Advantages of Anaerobic Digestion in Microbial Energy Conversion
One of the best things about anaerobic digestion is how easily it scales, making it a flexible component of the microbial energy conversion toolkit. Whether you’re running a small farm or managing a large municipal wastewater treatment plant, this technology adapts to your needs. Thanks to its modular nature, you can expand the system as your waste volumes grow, making it incredibly versatile.
Moreover, biogas is a real multitasker. It can be used directly for heating, electricity generation, or even as a transportation fuel when purified to biomethane. This versatility is what makes anaerobic digestion such a compelling option for anyone looking to reduce their carbon footprint and boost energy efficiency.
Example Case Studies of Microbial Anaerobic Digestion:
Germany’s Renewable Energy Program is a trailblazer in anaerobic digestion, especially on farms. The country operates ~9,500 farm-based biogas plants, which play a significant role in its renewable energy landscape. In 2021, the country’s 9,500 farm-based biogas plants produced 13 TWh of electricity. This accounted for 22% of the power supply typically provided by natural gas, and generating 2% of the nation’s heat supply. The catch, however, is that about 75% of the input for German biogas comes from energy crops. To enhance its impact, a shift towards using waste and biogenic by-products is essential. The REPower EU Plan aims to dramatically increase biomethane production by 2030.
The East Bay Municipal Utility District (EBMUD) in Oakland, California is another great example of anaerobic digestion at work. This facility converts sewage, food scraps, and industrial waste into biogas, which powers the entire treatment plant. In 2012, EBMUD became the first North American wastewater plant to produce more renewable energy than it consumes. This success was made possible by the installation of an energy-efficient gas turbine. EBMUD’s efforts generate about $3 million in annual electric power savings and sell excess energy back to the grid.
Microbial Fuel Cells in Microbial Energy Conversion
How Microbial Fuel Cells Work in Microbial Energy Conversion
Microbial Fuel Cells harness bacteria to convert organic waste into electricity. Inside a Microbial Fuel Cell, electrogenic bacteria break down organic compounds, releasing electrons. These electrons are captured by the anode and flow through an external circuit to the cathode, generating an electric current. Meanwhile, protons produced during this process pass through a membrane and combine with electrons and oxygen at the cathode to form water, completing the circuit and generating power.
Microbial fuel cells come in various designs. The single-chamber microbial fuel cell is the simplest, with both the anode and cathode housed in the same space, making it useful for low-power needs but with limited efficiency. Dual-chamber microbial fuel cells, which separate the anode and cathode with a membrane, improve efficiency but add complexity (See image above). Stackable microbial fuel cells link multiple cells in series or parallel to increase power output, making them more scalable for higher energy needs.
Scalability and Limitations of Microbial Fuel Cells in Microbial Energy Conversion
Microbial fuel cells offer potential for generating electricity from waste, but lower power outputs limit their scalability compared to technologies like anaerobic digestion or microbial electrolysis cells. This makes them better suited for small-scale, low-power applications rather than large-scale energy production.
Stackable microbial fuel cells can boost power output by connecting multiple units, making them more suitable for larger applications. However, scaling to industrial levels remains challenging due to the difficulty of maintaining efficient microbial activity and addressing power density limitations.
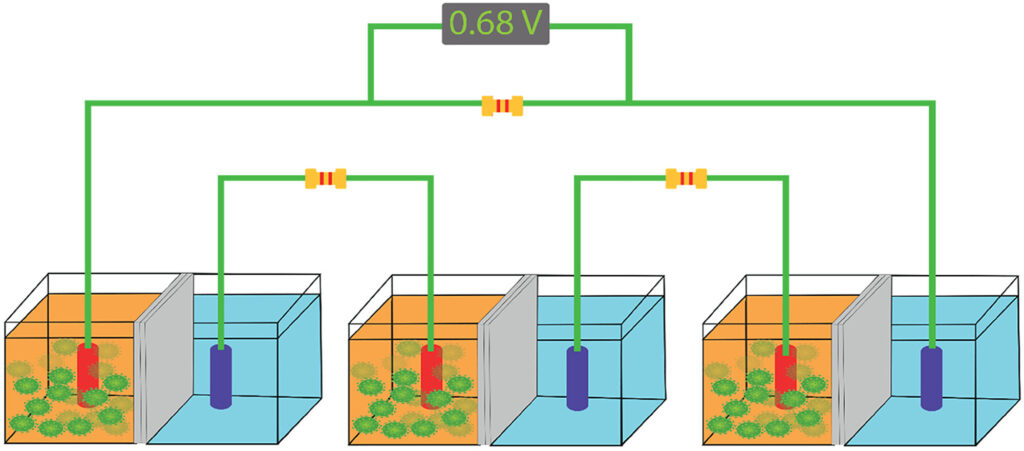
Applications of Microbial Fuel Cells in Microbial Energy Conversion
Microbial fuel cells are particularly well-suited for small-scale systems where steady, low-power electricity is needed. They really shine in applications like powering remote environmental sensors, especially in off-grid locations. These sensors often rely on minimal power, and microbial fuel cells can keep them running by converting organic matter from the surrounding environment, such as soil or sediment, into electricity—no batteries or external power sources required.
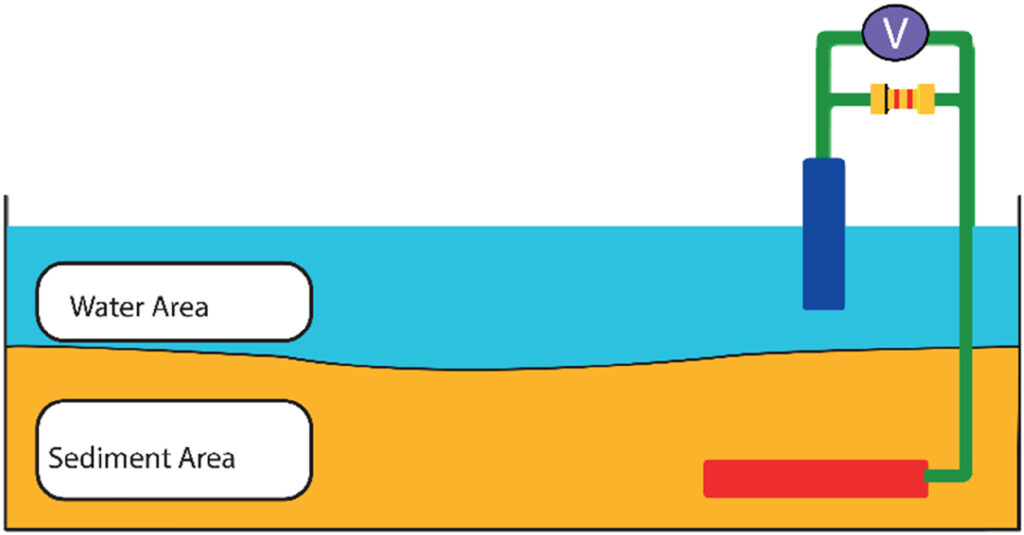
Beyond powering small devices, microbial fuel cells also play a role in wastewater treatment, making them a versatile tool in microbial energy conversion. Here, they offer a dual benefit: treating wastewater by breaking down organic pollutants and generating electricity at the same time. This makes microbial fuel cells a valuable technology for facilities aiming to improve energy efficiency while maintaining environmentally friendly practices.
Microbial Electrolysis Cells in Microbial Energy Conversion
How Microbial Electrolysis Cells Work
Microbial electrolysis cells advance microbial energy conversion by building on the principles of microbial fuel cells. While microbial fuel cells generate electricity directly from organic matter, microbial electrolysis cells go a step further by introducing an external voltage. This additional electrical input drives further electrochemical reactions, leading to the production of valuable fuels like hydrogen and methane.
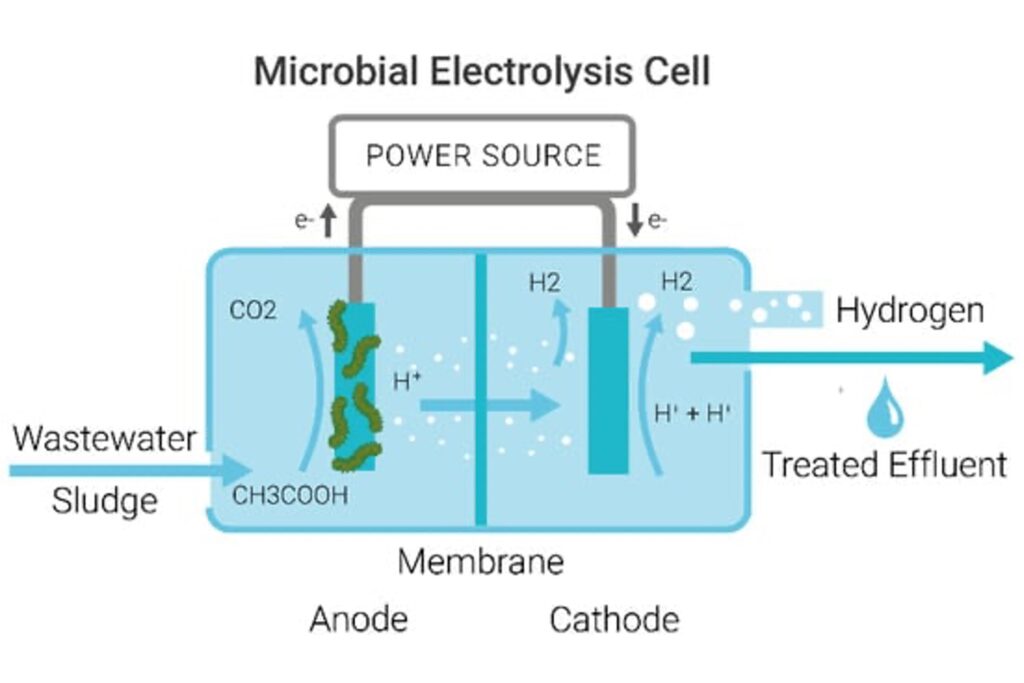
The process in an microbial electrolysis cell starts similarly to an microbial fuel cell. Electrogenic bacteria at the anode break down organic compounds, releasing electrons and protons. However, microbial electrolysis cells use the applied voltage to push these electrons toward the cathode, where different reactions occur. In hydrogen-producing microbial electrolysis cells, protons combine with electrons at the cathode to create hydrogen gas. In methane-producing microbial electrolysis cells, methanogens—specialized microorganisms—combine electrons and protons with carbon dioxide to produce methane, a process known as electromethanogenesis.
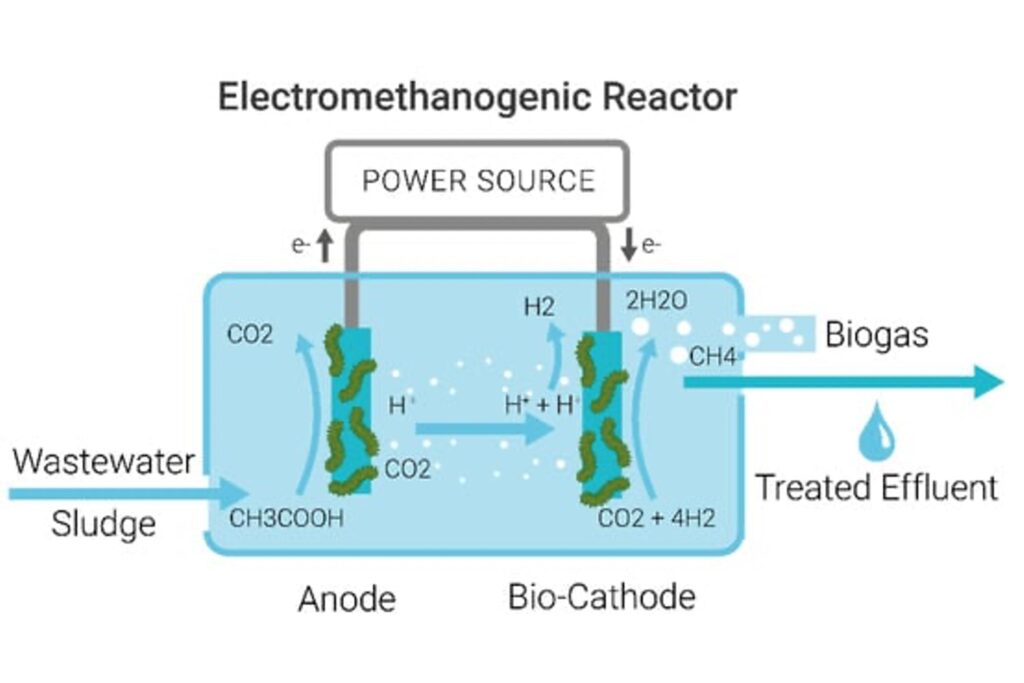
Why Adding Electricity Results in a Net Energy Gain
Adding electricity to microbial electrolysis cells may seem counterintuitive, but it actually results in a net energy gain. Although microbial electrolysis cells need an external voltage, they produce more energy in hydrogen or methane than they consume. This happens because of the energy stored within the chemical bonds of these gases. The applied voltage helps bacteria overcome activation energy barriers and efficiently convert organic matter into fuels.
For example, a small electrical input drives the conversion of protons and electrons into hydrogen, or carbon dioxide and electrons into methane. The energy content of hydrogen or methane far exceeds the electricity used, thanks to the energy stored in their molecular bonds. This efficiency is especially clear when methane is used in combined heat and power systems. These systems generate both electricity and heat, maximizing energy recovery. Even after accounting for the electricity used, microbial electrolysis cells can produce 20-30% more net energy than traditional anaerobic digestion.
Applications of Microbial Electrolysis Cells
Microbial electrolysis cells are versatile and find applications in various industries, particularly in wastewater treatment and bioenergy production. In wastewater treatment, microbial electrolysis cell break down organic pollutants while generating hydrogen or methane, making them valuable in industries like food and beverage production.
For instance, Cambrian Innovation’s EcoVolt Reactor, is an microbial electrolysis cell-based system inspired by NASA research that treats industrial wastewater while generating renewable energy. Initially designed to address challenges in space, this technology is now making a significant impact on Earth in industries like brewing and food processing.
The EcoVolt system uses microbial electrolysis cells to convert organic pollutants into methane, which is then used on-site as energy. This process not only reduces costs but also generates more energy than it consumes, making it energy-positive. Cambrian’s beverage-industry customers include Domaine Chandon, Tree House Brewing Company, and AB InBev.
Microbial Energy Conversion Method Comparison
Anaerobic digestion, microbial fuel cells (MFCs), and microbial electrolysis cells (MECs) each offer distinct benefits and challenges for microbial energy conversion, with differences in energy output, scalability, and cost-effectiveness.
Type |
Characteristics |
Energy Output |
Scalability |
Complexity |
Cost |
Best Suited For |
Anaerobic Digestion |
Anaerobic bacteria break down organic matter into biogas (methane and CO2) and digestate |
High; produces methane for electricity, heat, or upgraded to biomethane |
High; scalable from small farms to large municipal plants |
Moderate; well-established process but requires careful management of anaerobic conditions |
High initial costs, moderate operating costs; infrastructure-intensive |
Large volumes of organic waste; farms, municipal solid waste, industrial organic waste |
Microbial Fuel Cell |
Electrogenic bacteria break down organic matter, releasing electrons that are captured by an anode, with oxygen as the final electron acceptor |
Low; suitable for small-scale power generation |
Low to moderate; suitable for small applications |
Low; simple design, easier to maintain |
Low; cost-effective but limited scalability |
Small-scale, low-power applications; remote sensors |
Hydrogen-Producing Microbial Electrolyis Cell |
External voltage applied to drive hydrogen production at the cathode; utilizes hydrogen-producing bacteria |
High; produces hydrogen, suitable for storage and energy applications |
High; capable of scaling for industrial applications |
High; requires external voltage and careful management |
High; requires external energy input, more expensive materials |
Industrial-scale applications; energy-positive systems where hydrogen is a desired output |
Methane-Producing Microbial Electrolysis Cell |
External voltage applied to drive methane production at the cathode; utilizes electromethanogens to convert CO2 and electrons into methane |
High; produces methane, similar to biogas but with higher efficiency |
High; scalable for large-scale energy production |
High; involves more complex biochemistry and monitoring |
High; similar to hydrogen-producing MEC but with different outputs |
Industrial-scale applications; methane production for use as a renewable energy source |
Future Trends and Innovations in Microbial Energy Conversion
As the demand for sustainable energy grows, researchers are exploring new microbial systems and hybrid technologies. These approaches blend the strengths of anaerobic digestion, microbial fuel cells, and microbial electrolysis cells. One exciting development is the creation of hybrid systems. These setups combine the biogas production of anaerobic digestion with the electricity generation of microbial fuel cells. They also incorporate the hydrogen or methane production capabilities of microbial electrolysis cells. The goal is to maximize energy recovery from organic waste using multiple microbial processes in one integrated system.
But that’s only the beginning. Synthetic biology is making waves in microbial energy conversion. Scientists are modifying microbial metabolic pathways to make them more efficient at converting organic matter into energy. Whether it’s electricity, hydrogen, or methane, these engineered bacteria could lead to more adaptable and efficient energy systems. They can adjust to different waste streams and environmental conditions.
Another area of interest is bioelectrochemical systems. These systems expand microbial energy conversion by using bacteria to drive electrochemical processes. Bioelectrochemical systems may include advanced versions of microbial electrolysis cells and microbial fuel cells. They can also be designed to produce valuable byproducts like bioplastics, biofuels, and pharmaceuticals. With their flexibility, these systems could become key players in the future of waste-to-energy technologies.
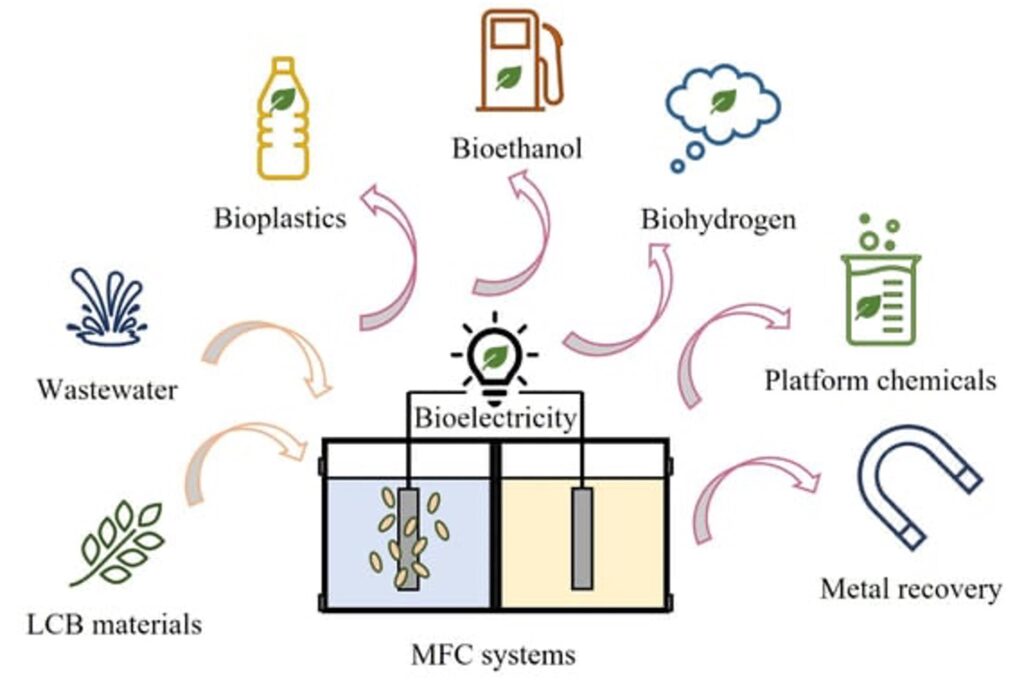
Conclusion
microbial energy conversion technologies show great promise in the transition to green energy. They offer versatile solutions for waste management and decentralized energy production. Each approach has its strengths. Anaerobic digestion excels in large-scale operations. Microbial fuel cells work well in smaller, low-power applications. Microbial electrolysis cells show strong potential for producing renewable fuels.
Looking ahead, microbial energy conversion will likely grow in importance. Advancements in hybrid systems and synthetic biology are on the horizon. These innovations could boost the efficiency and flexibility of waste-to-energy technologies. They are set to play a bigger role in industrial waste treatment, bioenergy, and chemical production.
Your Thoughts
Are there aspects of microbial energy conversion that surprised you or left you with questions? I would love to hear your thoughts! Please share your opinions on how these systems fit into the broader green energy landscape in the comments field at the bottom of the page.
Be sure to visit bleedingedgebiology.com next week for another “bleeding edge” topic!
Additional Materials
Videos
“A robot that eats pollution” TED Talk Jonathan Rossiter. Meet the “Row-bot,” a robot that cleans up pollution and generates the electricity needed to power itself by swallowing dirty water. Roboticist Jonathan Rossiter explains how this special swimming machine, which uses a microbial fuel cell to neutralize algal blooms and oil slicks, could be a precursor to biodegradable, autonomous pollution-fighting robots.
“How To Set Up a Microbial Fuel Cell” | Science Project This video shows how to set up a soil microbial fuel cell using a “Science Buddies” Microbial Fuel Cell kit.
“HAPPY MICROBES plus FOOD WASTE = CARBON FREE ENERGY” This Conversation with Teresa Keller. In this episode, Dr. Alex Lewis talks about Electro-Active Technologies, the company he co-founded in Knoxville, TN, and the initiative to produce carbon-free hydrogen energy. The sophisticated process relies on microbes eating massive amounts of food waste.
“The Living Fuel Cell Powered By Human Waste” | Fully Charged. Can human urine power a phone? Scientists at the University of the West of England (UWE) in Bristol are using human waste to generate electricity. Microbial fuel cells, or biological fuel cells, combine nature and engineering to create renewable energy. The UWE team is turning waste into clean power, with plans to trial the technology in refugee camps and developing countries. If successful, it could transform electricity access in remote areas.
Websites
Cambrian Innovation’s EcoVolt Technology Learn more about Cambrian Innovation’s EcoVolt system, which converts wastewater into energy using microbial technologies.
Bacterial Conversion in the U.S. Department of Energy Biological and Environmental Research Program. Lignocellulosic biomass, derived from plant materials like wood and crop residues, is a renewable resource for producing sustainable fuels and bioproducts. The Department of Energy’s Genomic Science Program (GSP) focuses on overcoming challenges in converting this tough material by engineering microbes through advanced genomic tools. These efforts aim to efficiently transform biomass into biofuels and bioproducts, providing a sustainable alternative to fossil fuels while enhancing the economic viability of the process.
SCI | Chemistry & Industry | Are microbial fuel cells ready to power the world? For decades, researchers have been trying to capture the electrons from bacteria to generate electricity in microbial fuel cells. So, are scientists finally starting to gain traction? XiaoZhi Lim reports
Books
“Applied Biotechnology for Emerging Pollutants Remediation and Energy Conversion” by B. Samuel Jacob, K. Ramani, et al. Springer 2023. Provides an in-depth look at how biotechnology is being harnessed to tackle pollutants like microplastics, hydrocarbons, and textile dyes that threaten ecosystems. The book also explores waste-to-energy conversion strategies, aligning with global goals for sustainable development.
“Sustainable Microbial Technologies for Valorization of Agro-Industrial Wastes (Novel Biotechnological Applications for Waste to Value Conversion)” by Jitendra Kumar Saini, Surender Singh, Lata Nain. CRC Press, 2022. The book covers key topics such as bioprocess development, environmental concerns, and scale-up challenges for commercial applications.
“Emerging Trends and Advances in Microbial Electrochemical Technologies: Hypothesis, Design, Operation, and Applications” by Asheesh Kumar Yadav, Pratiksha Srivastava, Md Tabish Noori, Yifeng Zhang. Elsevier 2024. Offers a comprehensive overview of the most recent developments in microbial electrochemical technologies, from lab research to practical applications. The book covers environmental uses like wastewater treatment, bioremediation, and resource recovery, while exploring new methods for electricity generation and emissions reduction.