Introduction
I’ve always been intrigued by how the fundamental laws of physics shape the living world. Recently, quantum biology has caught my attention as it examines how quantum mechanics applies to biological systems. The underlying premise is that phenomena like superposition and entanglement might influence life’s essential processes. Understanding these quantum effects in living organisms could transform our grasp of biology, and may help in the development of new technologies and medical treatments.
In this post, I will look at how quantum mechanics intersects with biology, examine key examples of this phenomenon, and consider the implications for science and technology. My aim is to introduce this fascinating but relatively obscure field and encourage curiosity about the quantum nature of life.
What Is Quantum Biology?
Definition and Scope
Quantum biology explores how quantum mechanics influences biological processes. I first encountered this field through the work of Johnjoe McFadden and Jim Al-Khalili, both professors at the University of Surrey—McFadden in molecular genetics and Al-Khalili in theoretical physics. Together, they co-authored Life on the Edge: The Coming of Age of Quantum Biology, where they investigate how quantum mechanics might drive complex biological functions like photosynthesis, enzyme activity, and potentially even consciousness.
Traditional biology relies on classical physics and chemistry to explain how organisms function. However, quantum biology suggests that some biological events cannot be fully understood without considering quantum effects. This field aims to bridge the gap between quantum mechanics and biological science, offering new perspectives on how life operates at the most fundamental level.
Historical Background of Quantum Biology
Schrödinger Suggests Quantum Biology
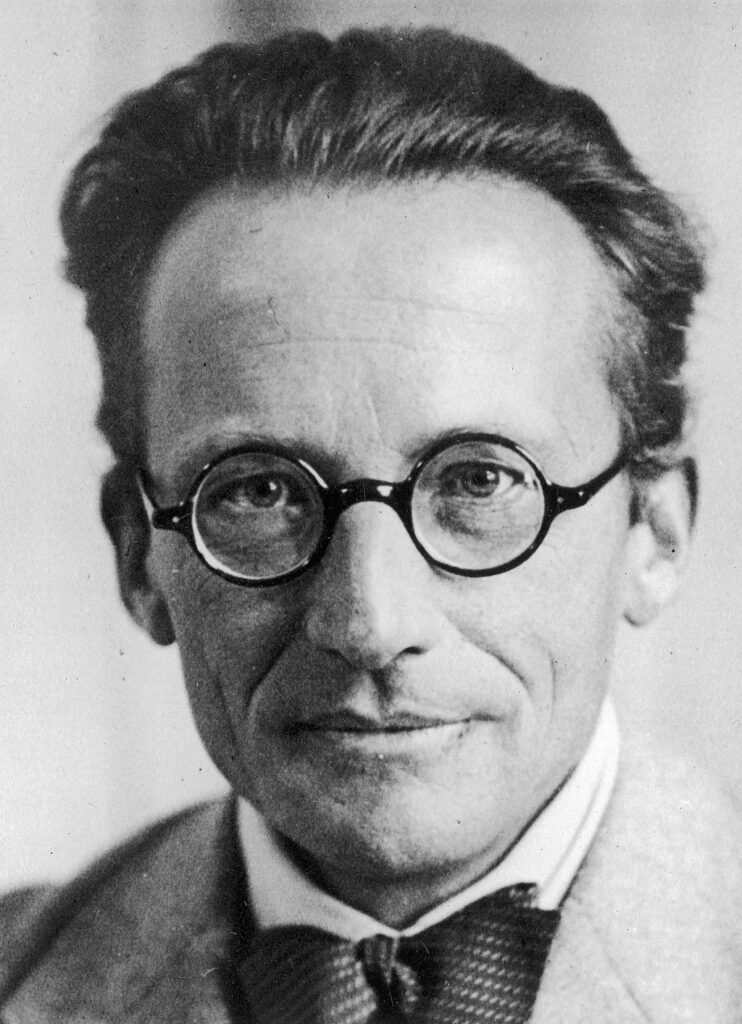
The concept of quantum biology isn’t new. In fact, in the 1940s, physicist Erwin Schrödinger—best known for his contributions to quantum mechanics, including the Schrödinger equation and the famous Schrödinger’s cat thought experiment—published a book titled What Is Life? In this influential work, Schrödinger speculated about the quantum principles underlying genetic information. He suggested that quantum mechanics might play a fundamental role in the processes governing life. His groundbreaking ideas challenged scientists to think beyond classical biology and consider the potential quantum nature of biological systems, sparking a new realm of inquiry.
For many years, however, quantum biology remained on the fringes of science due to the lack of experimental evidence. The complexity of biological systems, combined with the difficulty of detecting quantum effects at the biological level, meant that Schrödinger’s ideas were largely theoretical. Nevertheless, his work continued to inspire a small group of researchers. It wasn’t until the 21st century, when technological advancements allowed for more precise measurements, that significant breakthroughs began to emerge.
Pioneers in Experimental Quantum Biology
Greg Engel, a Professor of Chemistry at the University of Chicago, and Seth Lloyd, a Professor of Mechanical Engineering and Physics at MIT, are among those leading this new wave of discoveries. Engel’s research focuses on understanding quantum coherence in photosynthesis, exploring how plants and bacteria optimize energy transfer using quantum effects. Similarly, Lloyd has investigated quantum mechanics in biological systems, including photosynthesis, in an effort to determine how organisms maintain quantum states in challenging environments, such as warm and noisy conditions.
As a result of their efforts, experimental evidence of quantum phenomena in biological contexts, such as photosynthesis and enzyme activity, has come to light. Consequently, this has reignited interest in Schrödinger’s early speculations and opened up new possibilities for understanding how quantum mechanics might influence life processes. This renewed focus marks the beginning of an exciting new era for quantum biology.
Basic Principles of Quantum Mechanics Relevant to Biology
Understanding quantum biology requires a grasp of several key quantum mechanics concepts:
Superposition: In quantum mechanics, a particle can exist in multiple states simultaneously until it is observed. In biological systems, this principle may explain how molecules efficiently locate their targets within cells. For example, Graham Fleming, a Professor of Chemistry at the University of California, Berkeley, has extensively studied superposition in the context of energy transfer during photosynthesis.
Entanglement: This phenomenon occurs when particles become linked in such a way that the state of one instantaneously affects the state of another, regardless of the distance between them. Biologist Thorsten Ritz, a Professor at the University of California, Irvine, has explored how entanglement might help birds navigate by sensing Earth’s magnetic field, providing a quantum explanation for magnetoreception.
Quantum Tunneling: Quantum tunneling allows particles to pass through energy barriers that would be impossible to cross under classical physics. This effect is thought to play a critical role in enzyme catalysis and even DNA mutations. Professor Judith Klinman, in the chemistry department at the University of California, Berkeley, has pioneered research into quantum tunneling, particularly in enzymatic reactions, advancing our understanding of how biological systems overcome energy barriers.
Quantum biology posits that these effects—typically observed at the subatomic level—could have significant roles in biological processes. This concept challenges conventional scientific thinking and opens up exciting new avenues for exploration.
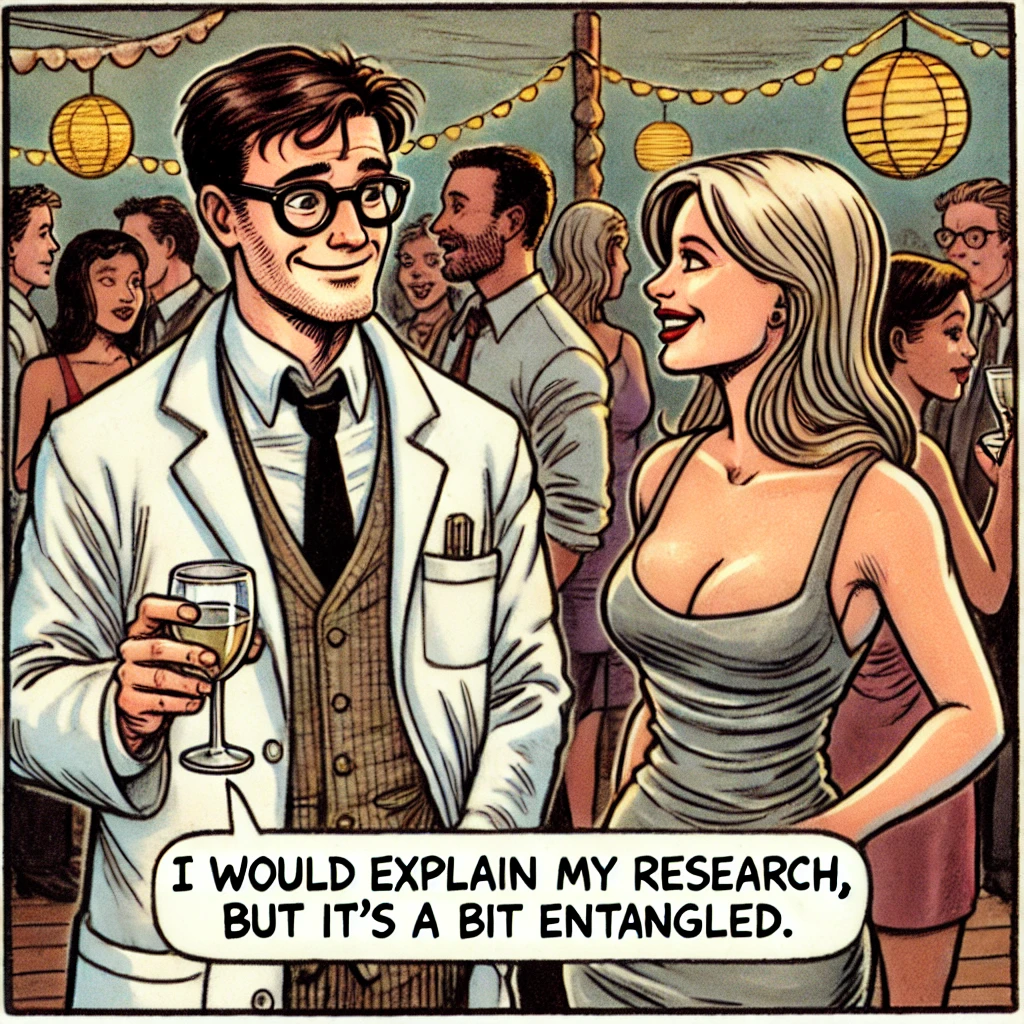
Critical Evaluation of Quantum biology
While the idea of quantum effects in biology is exciting, it’s essential to approach these theories critically. This is because some claims still lack strong experimental evidence and need more validation. Current research continues to explore how much quantum mechanics truly impacts biological systems.
Quantum Phenomena in Biological Processes
As I explored quantum biology more deeply, I found that quantum effects have been implicated in four major biological areas: enzyme catalysis, sensory processes, energy transference, and information encoding.
Enzyme Catalysis and Quantum Tunneling
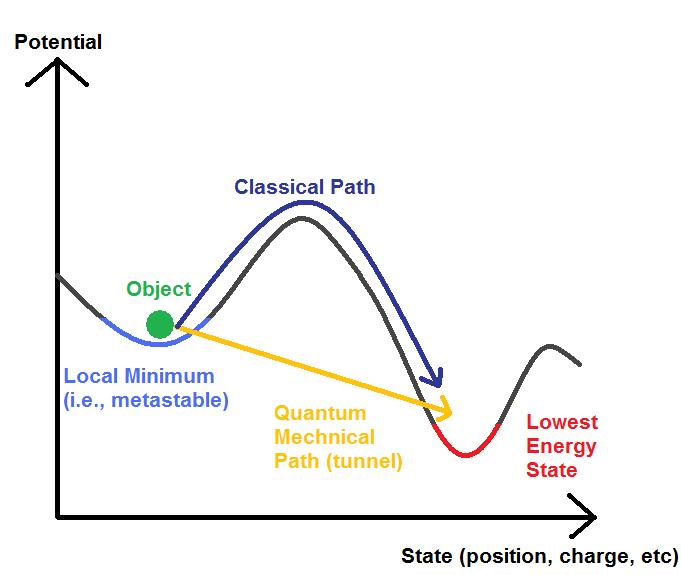
Enzymes are proteins that speed up chemical reactions in our bodies. Judith Klinman, has made groundbreaking discoveries in this area, providing strong evidence that quantum tunneling plays a critical role in enzyme function. Klinman, known for her pioneering work in enzyme catalysis, showed that quantum tunneling allows particles like electrons and protons to pass through energy barriers, even without the energy that classical chemistry would require. This research has transformed our understanding of how enzymes drive chemical reactions at the quantum level.
For example, studies on enzymes such as alcohol dehydrogenase have shown reaction rates faster than classical models predict. Quantum tunneling enables these particles to “tunnel” through energy barriers, making biochemical reactions more efficient. This quantum effect is crucial for essential processes like cellular respiration and DNA replication.
The Quantum biology of Sensory Processes: Olfaction and Vision
Olfaction: The Quantum Nose
Having worked in an olfaction lab for my postdoc, I am intrigued by Luca Turin’s quantum theory of odor detection. Turin, a biophysicist known for his research in olfaction, suggests a different way our noses detect scents. Rather than relying only on the shape of odorant molecules, he proposes we also detect differences in their vibrational frequencies.
According to Turin’s theory, when an odorant binds to an olfactory receptor, the resulting vibrational frequency could trigger electron tunneling within the receptor. This quantum process might explain how we perceive a vast range of scents with precision. Research supports the idea that animals can distinguish between molecules with similar shapes but different vibrational frequencies.
Vision: Quantum Effects in Photoreception
Our ability to see relies on the absorption of photons by retinal molecules in the eye. Interestingly, this process may involve quantum coherence, specifically through superposition. Research indicates that retinal receptorss detect single photons, suggesting that quantum processes efficiently convert light into neural signals.
Superposition allows retinal receptors to exist in multiple structural states simultaneously before photon absorption. This ability likely enhances the speed and efficiency of the receptor’s transition when interacting with light, triggering a nerve impulse. This process enables precise detection of light, even at very low intensities.
The Quantum Biology of Energy Transference: Photosynthesis and Mitochondria
Photosynthesis and Superposition
Photosynthesis is another area where quantum mechanics seems to play a significant role. This is suggested by the fact that plants and certain bacteria capture sunlight and convert it into chemical energy with remarkable efficiency. In 2007, Greg Engel and his team observed long-lived quantum effects in the Fenna-Matthews-Olson (FMO) complex of green sulfur bacteria at physiological temperatures.
The FMO complex is a light-harvesting structure in bacteria. It helps transport energy from chlorosomes to the reaction center for photosynthesis. Engel’s research suggests that superposition allows excitons—energy carriers produced by light—to explore several pathways at once. This ability lets them “choose” the most efficient route to the reaction center. As a result, the process reduces energy loss and maximizes the conversion of sunlight into chemical energy. This showcases how quantum mechanics may enhance biological efficiency.
Mitochondria and Electron Transport
Mitochondria, often called the powerhouses of the cell, generate energy through the electron transport chain. Researchers are exploring how quantum mechanics might explain the high efficiency of electron transport in these enzymatic pathways. In this case, quantum tunneling might play a role in the movement of electrons between transport chain complexes. This ensures cells maximize their energy production capabilities.
The Quantum Biology of Information Encoding: Magnetoreception and Mutation
The Quantum Biology of Magnetoreception in Birds
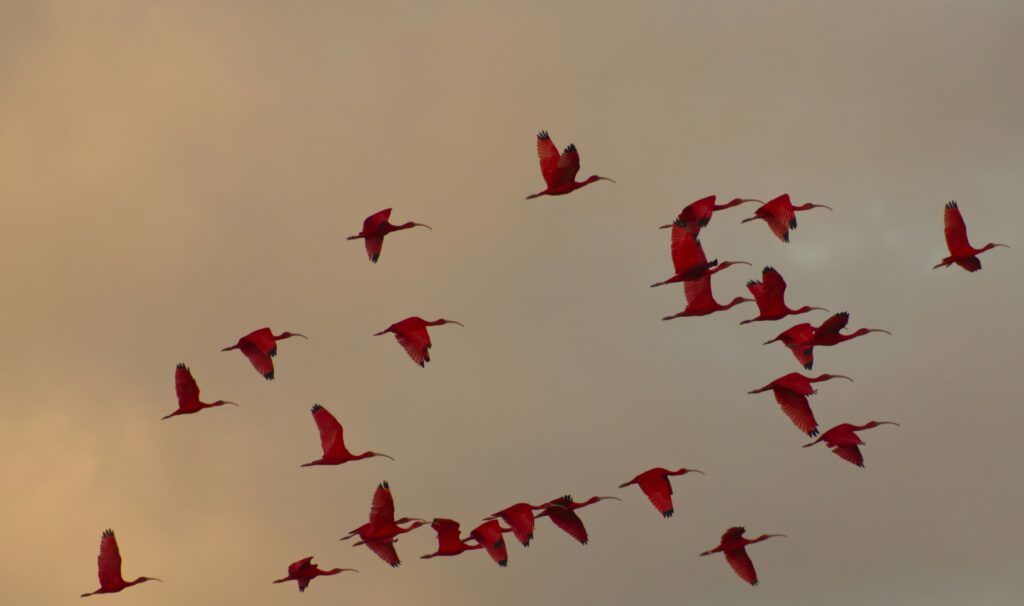
One of the enduring mysteries in biology is how migratory birds navigate long distances with such precision. A compelling hypothesis, first proposed by physicist Klaus Schulten at the University of Illinois and further developed by Thorsten Ritz, suggests that birds use quantum entanglement to sense Earth’s magnetic field—a process known as magnetoreception.
In this model, proteins known as cryptochromes, found in birds’ eyes, generate pairs of electrons with entangled spin states. These electron pairs, in turn, are sensitive to Earth’s magnetic field. As the magnetic field shifts, it influences the spin states, which then impact chemical reactions within the cryptochrome proteins. Consequently, this quantum mechanism could give birds an internal “compass,” allowing them to sense direction by detecting subtle changes in electron spin states driven by Earth’s magnetism.
Experiments have shown that when cryptochrome proteins are disrupted, birds lose their ability to navigate accurately. This supports the idea that cryptochromes are essential for magnetoreception and suggests that the quantum processes they facilitate may play a crucial role in how birds detect Earth’s magnetic field.
Quantum Effects in DNA Mutation
Quantum tunneling may also play a role in genetic mutations by allowing protons within DNA to shift between positions. As a result, this movement can lead to tautomeric shifts, which are changes in the hydrogen bonding patterns of DNA bases. These shifts can, in turn, cause replication errors. By understanding how quantum mechanics contributes to mutation processes, we could gain valuable insights into genetic disorders and the mechanisms driving evolution.
In fact, researchers like Johnjoe McFadden have discussed how quantum mechanics might influence mutation processes. Their work highlights the profound ways quantum phenomena may be intertwined with the very foundations of life.
Classical vs. Quantum Biology Models Comparison
(May need to scroll right)
Biological Process | Classical Explanation | Quantum Biology Explanation |
---|---|---|
Photosynthesis | Energy transfer occurs through classical hopping of excitons between pigment molecules in the light-harvesting complex. Efficiency is limited by thermal losses. | Energy transfer involves quantum coherence, allowing excitons to explore multiple pathways simultaneously through superposition. This leads to near-perfect efficiency by finding the most efficient route to the reaction center. |
Enzyme Catalysis | Enzymes lower the activation energy of reactions through induced fit and transition state stabilization, following classical over-the-barrier kinetics. | Enzymes facilitate reactions via quantum tunneling of particles like protons or electrons, allowing them to pass through energy barriers rather than over them, resulting in reaction rates higher than classical predictions. |
Bird Magnetoreception | Birds detect Earth’s magnetic field through classical magnetite-based receptors in their beaks or heads, aligning like tiny compasses to the magnetic field. | Birds use quantum entanglement in cryptochrome proteins in their eyes, forming radical pairs whose spin states are sensitive to magnetic fields, providing directional information through changes in chemical reactions influenced by Earth’s magnetism. |
Olfaction (Sense of Smell) | Odorant molecules are detected based on their shape fitting into olfactory receptors (lock-and-key model), triggering a neural response. | Olfaction operates via quantum tunneling where the vibrational frequencies of odorant molecules enable electrons to tunnel within receptors if the vibration matches, explaining the ability to distinguish molecules of similar shape but different smells. |
DNA Mutation | Mutations occur due to errors in DNA replication, chemical damage, or external factors like radiation, following classical chemical reaction pathways. | Quantum tunneling of protons in DNA bases leads to tautomeric shifts, causing mismatches during replication. This quantum effect contributes to spontaneous mutations beyond what classical chemistry would predict. |
Vision (Photoreception) | Photons cause isomerization of retinal molecules in photoreceptor cells through classical photoexcitation, triggering a neural signal for vision. | Vision involves quantum coherence where the photon’s energy is coherently transferred, enabling retinal molecules to respond to even single photons, suggesting quantum efficiency in the initial steps of phototransduction. |
Mitochondrial Energy Production | Electron transfer in the mitochondrial electron transport chain occurs through classical hopping between protein complexes, producing ATP with some energy loss. | Quantum tunneling facilitates efficient electron transfer between complexes in the electron transport chain, reducing energy loss and improving the efficiency of ATP production compared to classical expectations. |
Theoretical and Experimental Challenges in Quantum Biology
Investigating quantum phenomena within biological systems presents substantial challenges. Al-Khalili and McFadden have extensively discussed these obstacles, emphasizing the complexities involved in studying quantum effects in living organisms.
Maintaining Quantum states in Complex Biological Environments
Quantum effects such as superposition and entanglement are typically observed under controlled conditions at near-zero temperatures to prevent decoherence—the loss of quantum states due to environmental interaction. In contrast, biological processes occur in warm, dynamic environments where countless interactions can disrupt delicate quantum states. This makes it difficult to observe and measure quantum effects directly within living systems.
For instance, maintaining quantum coherence in biological molecules at physiological temperatures seems almost impossible. Yet, experiments by Greg Engel and Graham Fleming suggest that some organisms might have evolved mechanisms to protect quantum states. I find it fascinating that life could utilize quantum mechanics despite these challenging conditions.
Modeling the Quantum Phenomena of Biological Systems
Biological molecules are large and complex, involving numerous atoms and complex interactions. Modeling these systems requires simplifying assumptions that may overlook critical quantum effects. Unlike isolated particles studied in physics, biological molecules operate in environments where multiple variables influence outcomes.
This complexity makes it challenging to create accurate theoretical models that can predict quantum behavior in biological contexts. Researchers like Seth Lloyd have attempted to bridge this gap by using quantum computing simulations. Yet we will need more advanced computational tools to handle the intricacies of biological systems fully.
Technological Limitations of Quantum Biology
Detecting quantum phenomena at the molecular level requires highly sensitive instruments and advanced techniques. Current technology may not always provide the necessary resolution or may interfere with the biological processes being studied. For example, observing quantum coherence in photosynthesis demands ultrafast spectroscopy methods that can capture events happening in femtoseconds (one quadrillionth of a second).
Developing non-invasive tools that can monitor quantum states without altering the system remains a major hurdle. Scientists like Ahmed Zewail, an Egyptian-American chemist and Nobel Laureate, who pioneered the field of femtochemistry—the study of chemical reactions on extremely short timescales—have pushed the boundaries of what’s possible in understanding molecular processes. However, I believe we still have a long way to go before we can fully explore quantum biology without technological constraints.
Interdisciplinary Collaboration in Quantum Biology
Quantum biology sits at the intersection of physics, chemistry, and biology. As a result, progress in this field requires collaboration among scientists from different disciplines, each with their methodologies and terminologies. Bridging the gaps between these fields is essential but can be difficult due to differing perspectives and approaches.
For example, physicists might focus on mathematical models, while biologists emphasize empirical data. However, encouraging interdisciplinary research and communication is crucial for overcoming theoretical and experimental obstacles.
The Influence of the Observer Effect on Experimental Results in Quantum Biology
Quantum biology, in many ways, blurs the line between the observer and the observed. In quantum mechanics, before a system is measured, it can exist in an indeterminate state, described by a superposition of possibilities. A famous illustration of this is Schrödinger’s cat thought experiment.
In this hypothetical scenario, a cat is placed in a sealed box with a radioactive atom, a Geiger counter, a vial of poison, and a hammer. If the Geiger counter detects radiation from the decaying atom, the vial is broken, and the poison is released, killing the cat. However, if no radiation is detected, the cat remains alive. According to quantum mechanics, until we open the box and observe the system, the atom is in a superposition—it has both decayed and not decayed. Therefore, the cat is simultaneously alive and dead, existing in an indeterminate state.
This paradox demonstrates a key principle of quantum mechanics: systems can exist in multiple states simultaneously until they are observed or measured, which collapses the system into a single definite state. This is the essence of the observer effect—observation changes the state of the system.
Similarly, in quantum biology, our attempts to observe quantum phenomena in living organisms can alter the very processes we are studying. This makes it challenging to fully understand how quantum mechanics functions in biological systems. The following examples illustrate how the observer effect presents obstacles in quantum biology, affecting our ability to study and interpret quantum phenomena in living systems.
Quantum Coherence in Photosynthesis
When studying quantum superposition in photosynthesis, scientists use ultrafast laser spectroscopy to observe energy transfer within light-harvesting complexes. However, the act of measurement collapses the superposition of states of the excitons (energy carriers), altering the system’s natural quantum behavior.
Quantum Entanglement in Magnetoreception
Similarly, in researching magnetoreception in birds, attempts to measure the entangled electron spins in cryptochrome proteins result in the collapse of their entanglement. Once again, measuring the system changes its quantum state, making it challenging to directly study how quantum entanglement might aid in navigation.
Strategies to Overcome Challenges
The scientific method relies on observation, experimentation, and replication. However, measuring quantum states inherently alters them, making it hard to observe systems without influencing their natural behavior. While the observer effect complicates experimentation, it does not fundamentally undermine the scientific method.
For instance, scientists facing similar issues in quantum physics have developed indirect measurement techniques, theoretical models, and statistical methods to account for these challenges. In quantum biology, we can adopt similar strategies, such as using non-invasive measurement methods or relying on computational models to predict quantum behaviors in biological systems.
Skepticism and Acceptance in the Scientific Community
The idea that quantum mechanics plays a role in biological processes is still met with skepticism by some in the scientific community. These reservations can impact funding opportunities and the publication of research findings.
Overcoming this barrier involves providing robust experimental evidence and developing theoretical frameworks that can withstand scrutiny. Researchers like Luca Turin, who proposed the quantum theory of olfaction, have faced difficulties in gaining acceptance. I believe that as more empirical data emerges, the scientific community will become more receptive to the possibilities of quantum biology.
Implications and Future Directions
Revolutionizing Medicine and Drug Development
Quantum biology has the potential to revolutionize both medicine and technology. For example, by understanding quantum effects in enzymes, Peter Hore, a Professor of Chemistry at the University of Oxford, is exploring how these principles could help design drugs that interact more precisely with biological targets. Recognizing the role of quantum tunneling in enzyme reactions, in particular, could lead to pharmaceuticals that better regulate metabolic processes.
Advancements in Medical Diagnostics
In medical diagnostics, quantum sensors inspired by biological systems could detect diseases at much earlier stages. Michael Biercuk, a Professor of Quantum Physics and Quantum Technology at the University of Sydney, is pioneering quantum coherence-based sensors capable of identifying minute changes in biological tissues. These advancements could dramatically improve the accuracy of screenings for conditions such as cancer or neurological disorders.
Impact on Gene Therapy
Quantum biology’s influence extends to gene therapy as well. Vlatko Vedral, a Professor of Quantum Information Science at the University of Oxford, is studying how quantum mechanics impacts DNA mutations. By understanding how quantum effects contribute to genetic changes, researchers could develop methods to prevent harmful mutations or correct them before they lead to disease.
Quantum Biology and Computing
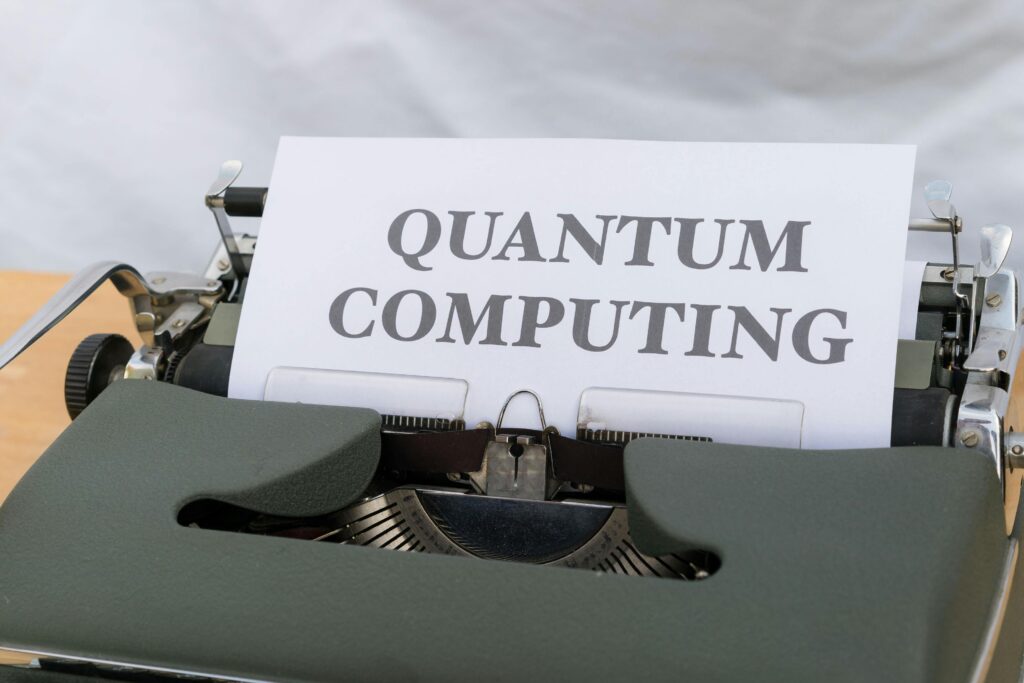
Quantum biology may provide key insights for the development of quantum computers. Biological systems have evolved to maintain quantum coherence in warm, complex environments—precisely the challenge quantum computing faces today. For example, the way plants preserve quantum states during photosynthesis has inspired Seth Lloyd to explore new methods for protecting qubits from decoherence. By mimicking these biological processes, researchers hope to create more stable and efficient quantum computers
Quantum Biology, Consciousness and Artificial Intelligence
The involvement of quantum effects in consciousness is purely speculative at this point. However, in my previous post on consciousness I discussed Orchestrated Objective Reduction (Orch-OR) theory, developed by Stuart Hameroff, a Professor of Anesthesiology and Psychology at the University of Arizona, along with Roger Penrose, a Professor Emeritus of Mathematics at the University of Oxford. This theory proposes that consciousness arises from quantum processes within microtubules in brain synapses. In the realm of artificial intelligence, understanding quantum information processing in the brain could open new possibilities. If this is found to occur in our mentition, it could lead AI systems that more closely mimic human cognition by incorporating quantum principles.
Advancing Interdisciplinary Collaboration
Progress in quantum biology depends on collaboration across multiple scientific disciplines. Therefore, encouraging partnerships between physicists, biologists, chemists, and engineers can accelerate discoveries and innovations. Initiatives like the Quantum Biology Network, involving scientists such as Jim Al-Khalili and Johnjoe McFadden, are fostering interdisciplinary research.
Educational programs that integrate these fields will play a crucial role in training the next generation of scientists to think beyond traditional boundaries. For example, universities are starting to offer courses and degrees that combine quantum physics and biological sciences, which is a promising development.
Future Research Directions
As technology advances, the potential to explore quantum effects in a wider range of biological processes grows steadily. In fact, areas such as neurobiology, genetics, and ecology may soon uncover new quantum mechanisms that influence life at its core.
However, one major challenge in quantum biology is developing effective experimental methods. For instance, these methods must allow us to observe and manipulate quantum states in biological systems without causing disruptions. As tools and techniques improve, we could unlock new insights into how quantum effects shape processes like enzyme activity and brain function. As a result, we may ultimately reshape our understanding of life.
Looking ahead, the future of quantum biology is full of promise. As research progresses, we might witness breakthroughs that transform our understanding of both biology and quantum physics, paving the way for advancements in technology, medicine, and beyond.
Some Philosophical Implications of Quantum Biology
The Nature of Free Will and Consciousness
Reflecting on quantum biology brings up profound philosophical questions. For instance, if quantum processes are indeed involved in conscious thought, this could reshape our understanding of consciousness.
One area this might have an impact is on our concepts of free will and determinism. In classical physics, systems are largely deterministic—given initial conditions, future states can be predicted. However, quantum mechanics introduces inherent randomness and probabilities. If quantum processes influence neural activity, this randomness could affect our decision-making.
For example, consider the idea that quantum fluctuations might impact neurotransmitter release in synapses. These tiny variations could potentially alter neural signaling pathways, leading to different thoughts or actions. This possibility intrigues me because it adds complexity to our understanding of human agency and responsibility.
Quantum Biology Reveals the Interconnectedness of Life and the Universe
Another fascinating idea is the interconnectedness implied by quantum entanglement. That is, if particles within our bodies are entangled with those in the environment, it suggests a deep connection between all living things. While this connection might not have immediate practical implications, it prompts us to consider the philosophical notion that all matter is fundamentally linked at the quantum level.
Philosopher and theoretical physicist Fritjof Capra, has explored this idea in depth. In his book The Tao of Physics, Capra argues that quantum mechanics supports a more interconnected understanding of nature. This perspective promotes a holistic view of life and our place in the universe, potentially shaping how we think about environmental conservation and our relationships with other organisms.
Conclusion
Quantum biology has the potential to reshape our understanding of life at the molecular level and beyond. By incorporating principles of quantum mechanics, we may gain an understanding of processes that classical biology struggles to fully explain. Moreover, this approach provides explanations that were previously unimaginable. For example, understanding the quantum influences in neural processes could revolutionize neuroscience, providing fresh insights into consciousness and cognition. Quantum biology challenges us to re-examine established biological concepts and consider the deeper physical laws that underlie their mechanics.
Quantum biology is still in its early stages, but it’s clear that the intersection of quantum physics and biology will be an exciting frontier of scientific discovery. Stay curious—quantum biology may very well hold the key to unlocking the next generation of breakthroughs in our understanding of life.
Your thoughts?
Quantum biology is a fascinating and rapidly emerging field, but it raises as many questions as it answers. What do you think about the idea that quantum mechanics might be essential to understanding life processes like photosynthesis, bird navigation, or even consciousness? Could quantum phenomena help unlock new medical advancements or fuel breakthroughs in computing? Share your thoughts, questions, or ideas at the bottom of the page.
Be sure to visit bleedingedgebiology.com next week for another “bleeding edge” topic!
Further Reading and Resources
To deepen your understanding of quantum biology, here are some key books, articles, and lectures by the scientists mentioned in this post:
Books
Erwin Schrödinger “What Is Life? The Physical Aspect of the Living Cell” (1944). Schrödinger explores how quantum physics might underpin genetic information, laying the groundwork for quantum biology.
Jim Al-Khalili and Johnjoe McFadden “Life on the Edge: The Coming of Age of Quantum Biology” (2014).
An accessible introduction to quantum biology, discussing how quantum mechanics influences biological phenomena.
Stuart Hameroff and Roger Penrose “Consciousness in the Universe: A Review of the ‘Orch OR’ Theory” (2014). This work examines the possibility of quantum processes contributing to consciousness.
Fritjof Capra “The Tao of Physics: An Exploration of the Parallels Between Modern Physics and Eastern Mysticism” (1975). While not focused on quantum biology, this book offers insights into the interconnectedness of all things at the quantum level.
Articles and Research Papers
Yuan-Chung Cheng, and Graham R. Fleming “Dynamics of Light Harvesting in Photosynthesis” Annual Review of Physical Chemistry (2009) This review emphasizes experimental observations of long-lasting quantum coherence in photosynthetic systems and the implications of quantum coherence in natural photosynthesis.
Thorsten Ritz, Salih Ahmad, and Klaus Schulten “Resonance effects indicate a radical-pair mechanism for avian magnetic compass” Nature (2004) Research supporting the idea that birds use quantum entanglement for navigation.
JP Klinman, A Kohen “Hydrogen tunneling links protein dynamics to enzyme catalysis” Annual review of biochemistry, 2013 This article explores how quantum mechanical hydrogen tunneling during enzymatic C-H bond cleavage reveals the critical role of protein dynamics in optimizing catalysis, with implications for enzyme activity, drug design, and biomimetic catalyst development.
Luca Turin “A spectroscopic mechanism for primary olfactory reception” Chemical Senses (1996) The original paper proposing the quantum vibration theory of olfaction.
Online Lectures
Jim Al-Khalili |“How quantum biology might explain life’s biggest questions” | TEDGlobalLondon 2015 Jim Al-Khalili rounds up the extremely new, extremely strange world of quantum biology, where something Einstein once called “spooky action at a distance” helps birds navigate, and quantum effects might explain the origin of life itself.
Johnjoe McFadden | “Quantum Biology Explained” |Chasing Consciousness Podcast|Discusses the emerging field of quantum biology, exploring how recent research into quantum coherence in avian navigation, quantum tunneling in DNA, and enzymatic reactions challenges the long-held belief that biological systems are too warm and wet to support quantum effects, with implications for life and evolution.
“Quantum Biology: The Hidden Nature of Nature” | World Science Festival This podcast explores how quantum physics may influence bird navigation, photosynthesis, and even smell, as experts in quantum biology discuss its potential to revolutionize fields like energy and quantum computing.